How 3D Printing is Accelerating Tissue Engineering Research at The University of Sheffield
Despite being the most sophisticated machine on earth, the human body is limited in its ability to repair itself. If damaged through injury or disease, most of our tissues and organs are unable to regenerate to their original state.
Our vascular network is made up of a web of intricate tubes that are responsible for transporting nutrients and oxygen around the body. Damage or blockages to this network can reduce vital blood supply and can cause major complications like strokes or heart attacks.
Conventional treatments for cardiovascular conditions rely on autografts. This involves harvesting a blood vessel from a less vital part of the patient’s body and using this to repair or bypass a more important damaged or blocked vessel. For example, harvesting a vein from the patient’s leg and using it to bypass a coronary artery that supplies blood to the heart.
However, the availability of autografts is limited and obtaining them requires invasive surgery. Synthetic vascular grafts made from polymer materials are also available, although these are prone to infection and blood clotting, especially in smaller diameter vessels. This is where tissue engineering fits in.
Tissue engineering refers to the development of technologies to grow replacement tissues and organs in the laboratory for use in treating injury and disease. The technique uses cells taken from the patient requiring treatment, which eliminates the possibility of rejection by the immune system - a major issue in conventional organ transplant procedures.
Over the last few years, tremendous advances have been made towards producing tissue-engineered blood vessels, but researchers have only been able to construct simple straight tubes. The vascular network is complex, containing bends, tapers and branches and recreating these shapes remains a challenge.
Dr. Sam Pashneh-Tala from the University of Sheffield is rising to this challenge. His research uses high-precision desktop stereolithography (SLA) 3D printing to enable the production of tissue-engineered blood vessels with a variety of geometries. This will allow for patient-specific vascular graft designs, improved surgical options and provides a unique testing platform for new vascular medical devices for those suffering from cardiovascular disease - which is currently the number one cause of death worldwide.
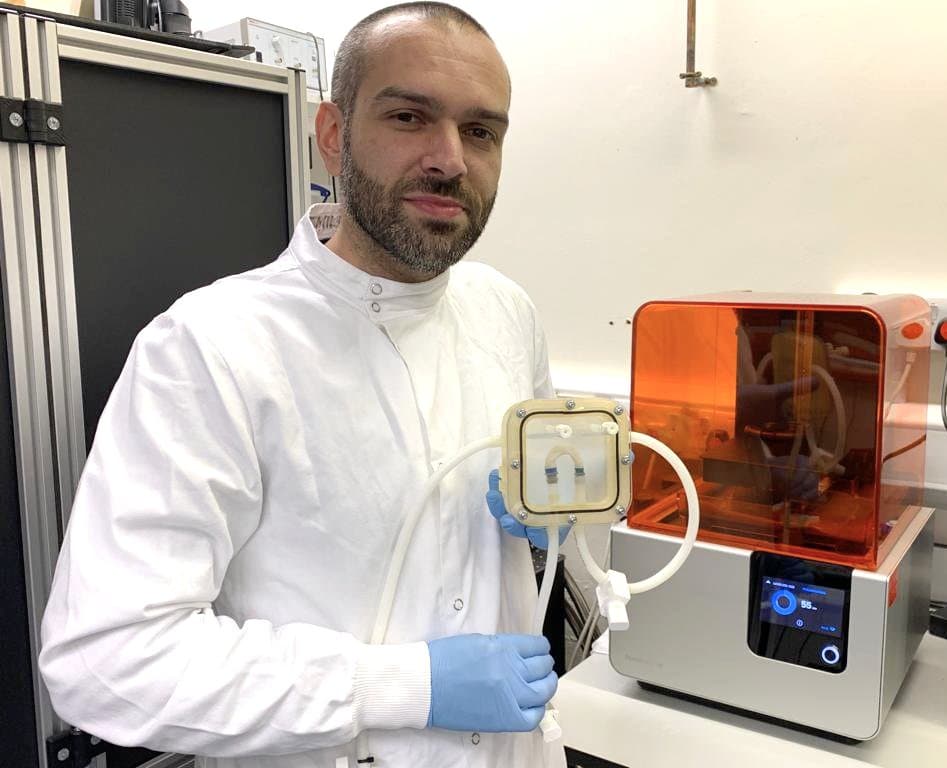
Bioprinting and 3D Printing in Tissue Engineering
The flexibility and precision of 3D printing as a manufacturing technique naturally lends itself to tissue engineering. The tissues that make up our bodies are an intricate 3D arrangement of cells and structural proteins, but these are also highly variable, as everyone’s body is unique.
Methods of directly 3D printing with cells to form tissues and organs are being developed. This may sound like fiction, but the reality is it’s happening right now. These “bioprinting” techniques can reproduce the geometry of natural tissue. However, recreating its mechanical and biological performance is more difficult.
An alternative approach is to direct the growth of cells into forming the required tissue in the lab. Most cells grown in vitro will not naturally arrange themselves into 3D structures. To achieve this, the cells can be grown on a scaffold, which provides a template of the shape required. For example, a tubular scaffold can be used to produce a blood vessel. The cells will multiply and cover the scaffold, taking its shape. Over time, the scaffold breaks down, leaving the cells now arranged into the shape of the target tissue.
Dr Pashneh-Tala uses a hybrid approach, in which 3D printing enables the production of tissue-engineering scaffolds for growing blood vessels with unique geometries. These scaffolds are then seeded with cells and matured in culture in the lab.
To date, vascular tissue engineering research has focused on producing straight vessels. These are not able to accurately mimic the vessel architecture found in the body and place limitations on vascular surgeons. 3D printing is the perfect technology to tackle this problem, as Dr Pashneh-Tala is able to create organic, custom shapes at no extra cost.
“My differentiator is that I’m able to create blood vessels with geometries that are more closely matched to those found in the body. This offers the potential for improved surgical options and even patient-matched blood vessel designs. Without access to high-precision, affordable 3D printing, creating these shapes would not be possible.”
Dr Sam Pashneh-Tala, Research Fellow, The University of Sheffield
Scaffold Manufacturing: At a Glance
- Specify the scaffold shape and create a CAD design of the scaffold mould negative
- 3D print the scaffold mould negative using desktop SLA technology
- Cast the 3D printed moulds with silicone and cure to obtain the scaffold moulds
- Assemble the silicone scaffold moulds
- Inject the scaffold material (biodegradable polymer emulsion) and photocure to create a solid, porous scaffold
- Sterilise the scaffold and seed with cells
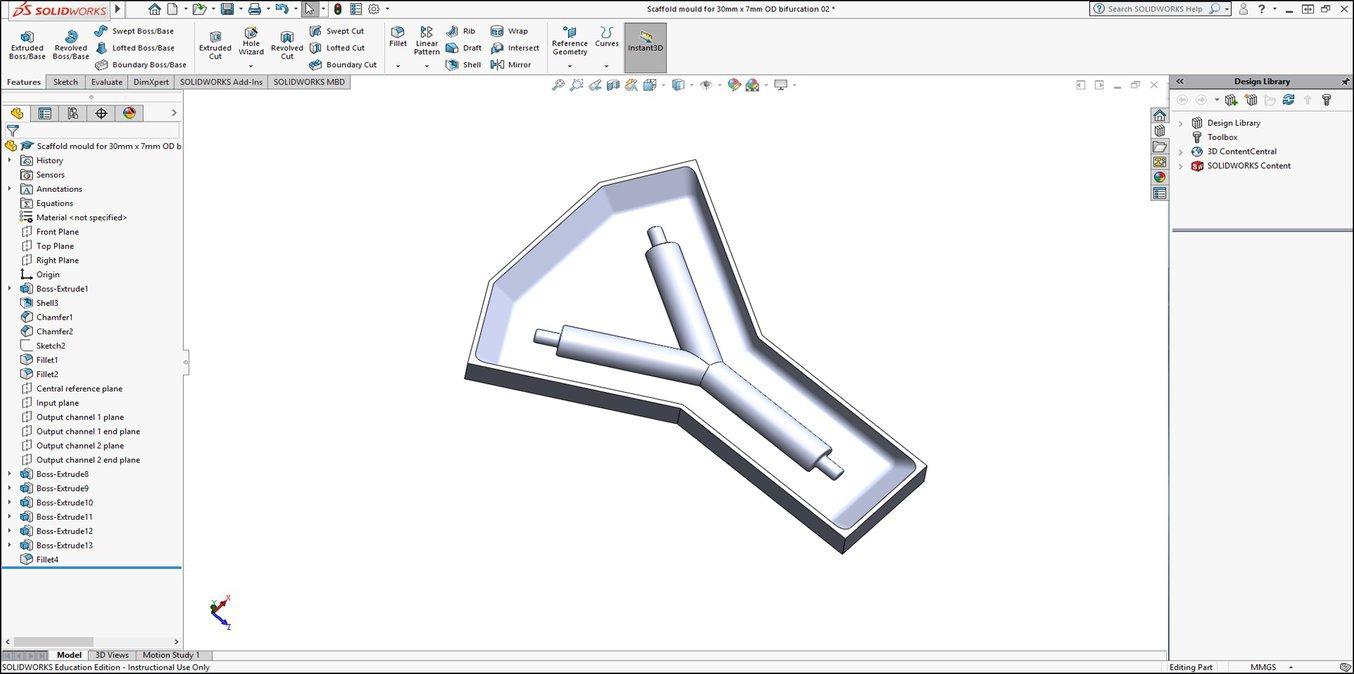
1. Specify the Scaffold Shape and Create a CAD Design of the Scaffold Mould Negative
Dr Pashneh-Tala uses 3D printing and silicone casting to produce the moulds for manufacturing his tissue engineering scaffolds. Working backwards from the desired scaffold design, a negative of the mould that will shape the scaffold is designed with SolidWorks® CAD software.
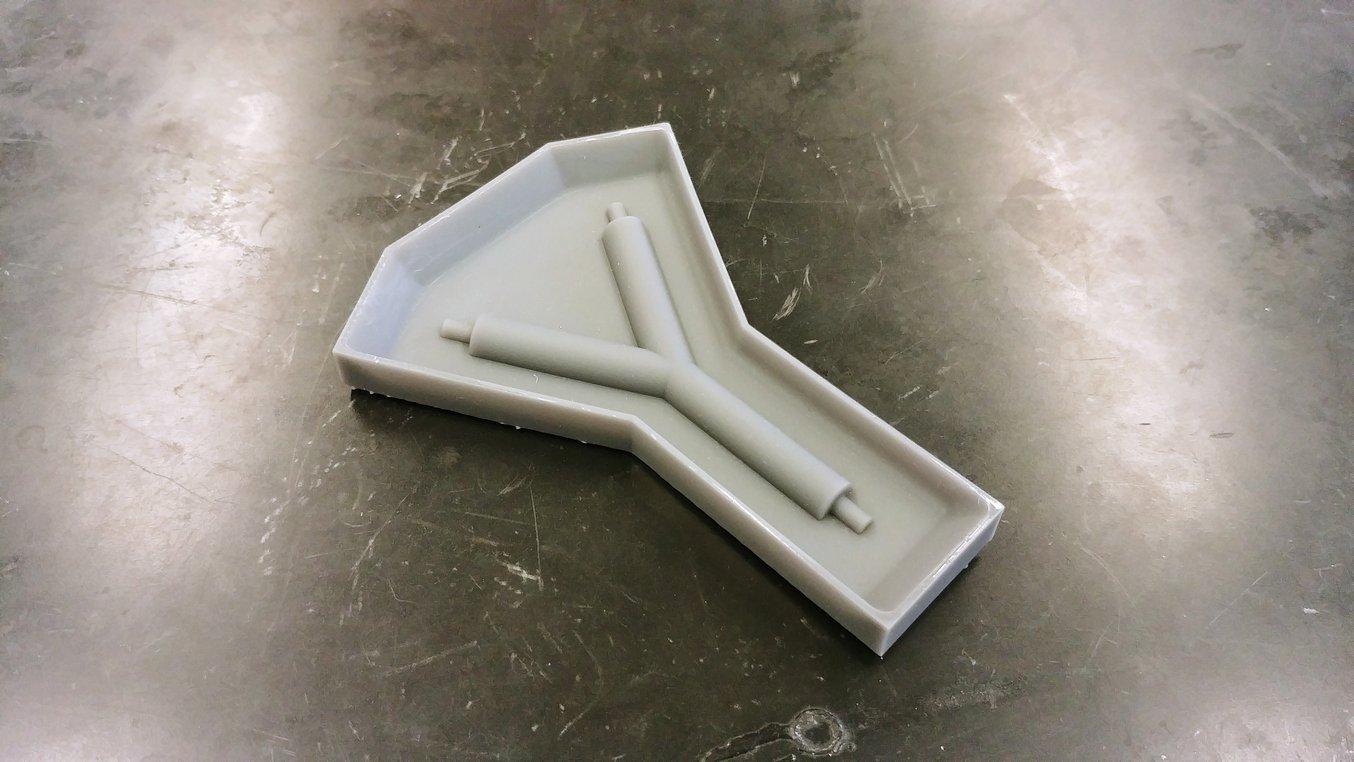
2. 3D Print the Scaffold Mould Negative Using Desktop SLA Technology
The CAD design is 3D printed in Dr Pashneh-Tala’s lab using his Formlabs SLA printer and Grey resin. The alternative would be sending the design to a third party service provider to be 3D printed or machined. This would add weeks to the development cycle of the tissue-engineered vessels and ultimately make the research unfeasible within his laboratory.
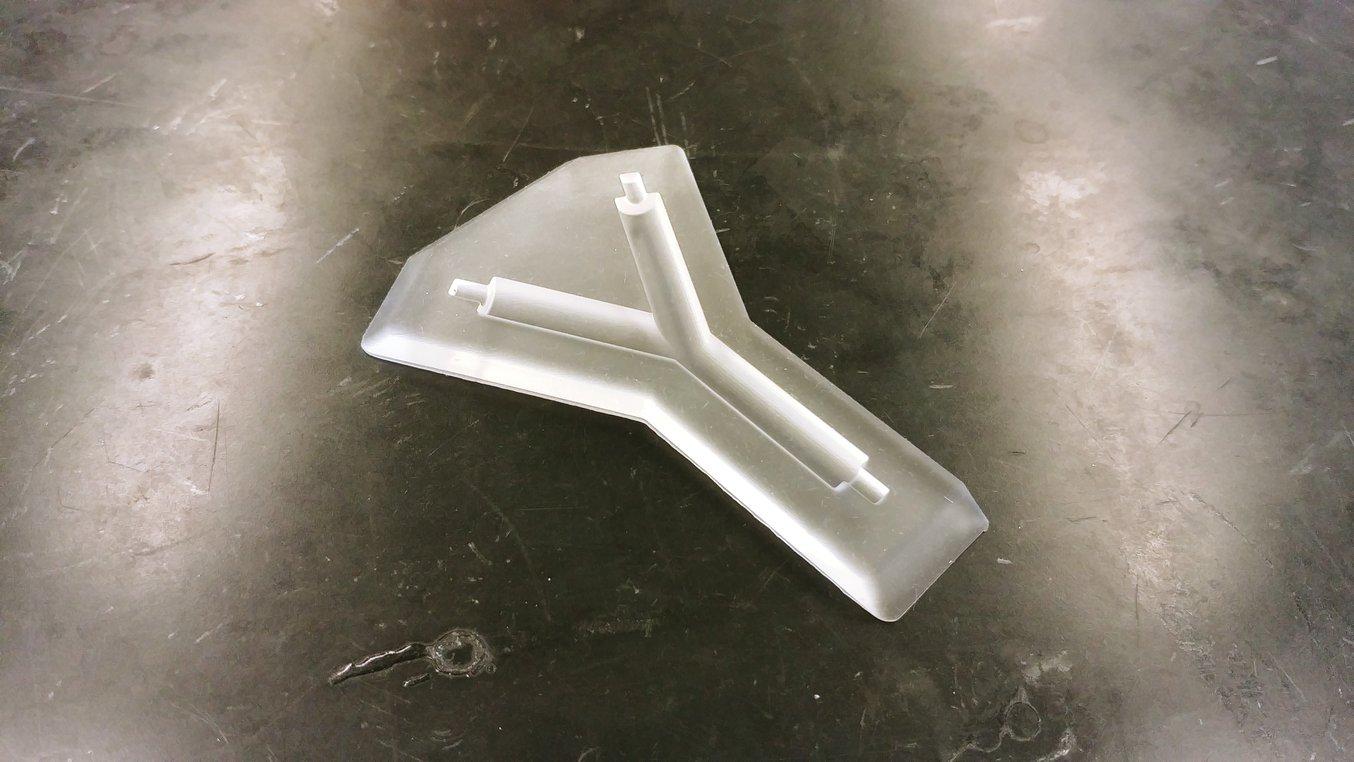
3. Cast the 3D Printed Moulds with Silicone and Cure to Obtain the Scaffold Moulds
The 3D printed mould negatives are then cast with silicone. The silicone is cured in an oven at 60°C and then removed. This produces the silicone moulds that will be used to shape the scaffold.
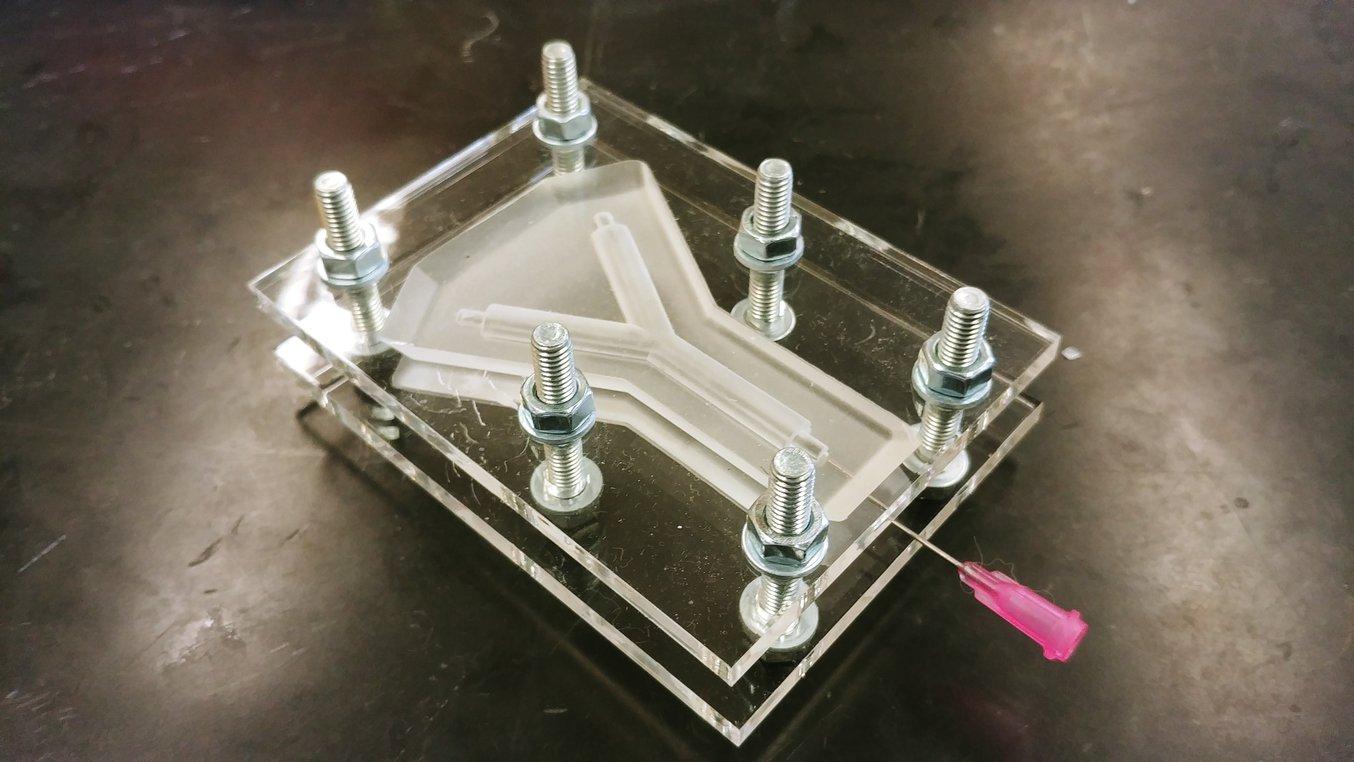
4. Assemble the Silicone Scaffold Moulds
Multiple silicone moulds are assembled together to correctly produce the scaffold shape. Silicone moulds are required to shape the outer surface of the scaffold, and also to produce the channel (lumen) that will run through its centre.
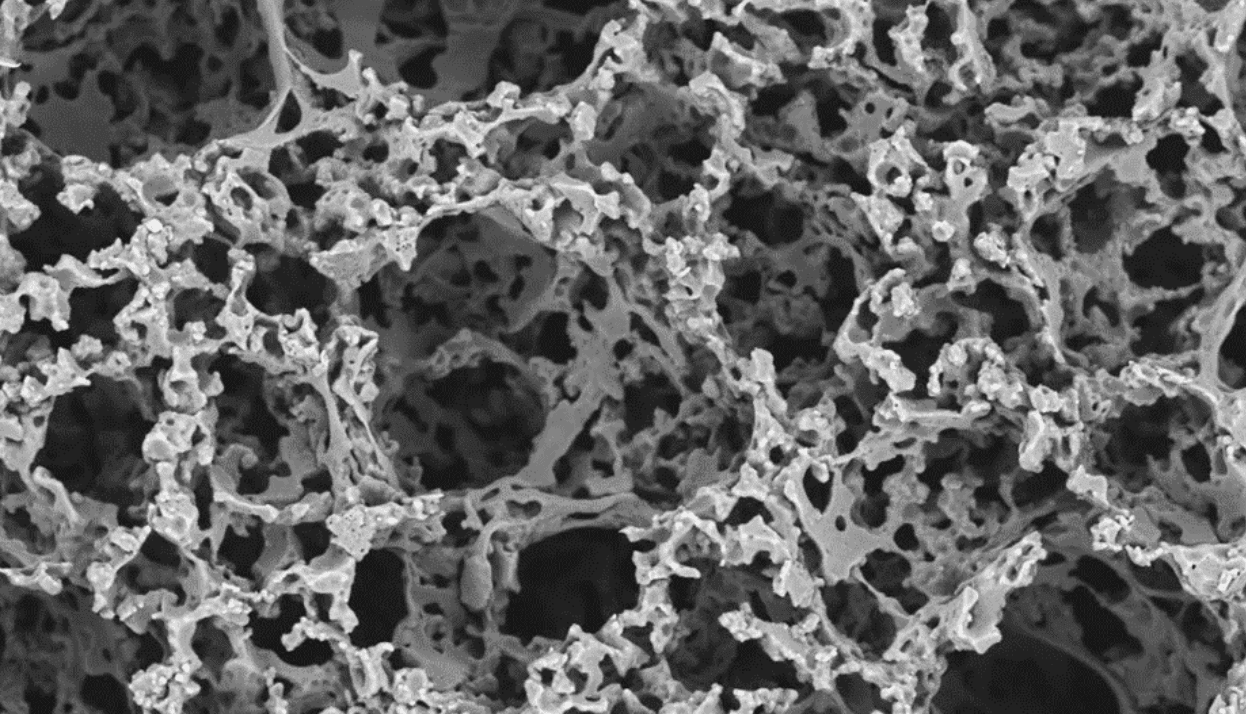
5. Inject the Scaffold Material (Biodegradable Polymer Emulsion) and Photocure to Create a Solid, Porous Scaffold
The mould cavity is filled with a custom biodegradable polymer emulsion which was developed at The University of Sheffield by Dr-Pashneh-Tala and his team. This flexible and biocompatible polymer, abbreviated to PGS-M, is ideal for growing cells on. PGS-M is also photocurable, which allows the liquid polymer emulsion to be transformed into a solid when exposed to UV light. After photocuring, the mould is opened to reveal a porous scaffold with microscopic holes that will enable cells to grow into it during in vitro culture.
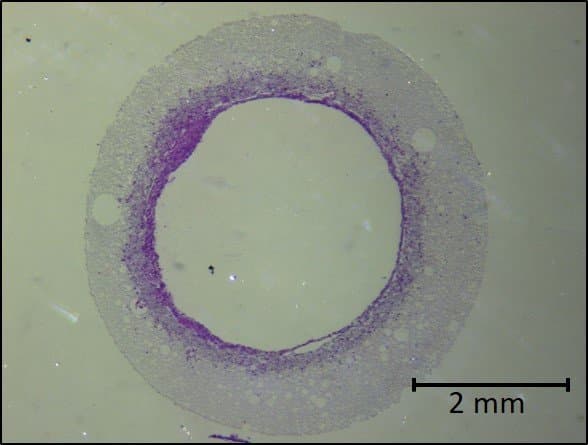
6. Sterilise the scaffold and seed with cells
Lastly, following sterilisation, Dr Pashneh-Tala cultivates the cells around the scaffold. Over time, the scaffold structure will degrade and disappear, leaving just the cells in the desired shape.
Dr Pashneh-Tala has also recently begun exploring the use of Formlabs Elastic Resin as a replacement for the cast silicone. With the aim to allow the production of the moulds to produce his scaffolds directly, streamlining the manufacturing process and reducing development time.
3D Printing a Bioreactor Chamber for Growing a Tissue-Engineered Aorta
Once the tissue engineering scaffold has been seeded with cells, it must be cultured in the lab in order to mature into the required tissue. This process is performed inside a bioreactor, a chamber that contains the developing tissue and can reproduce the internal environment of the body.
Mimicking the environmental factors of the body is instrumental in guaranteeing the success of laboratory tissue culture. The bioreactor contains liquid growth medium which nourishes the cells of the developing tissue, just like blood. The bioreactor is operated inside an incubator, which reproduces the body’s natural temperature of 37 °C, and it also isolates the tissue in a sterile environment, free from potential infection or contamination.
For the optimum development of tissue-engineered blood vessels, it’s also important to recreate the mechanical environment that the vessels experience in the body. To mimic blood flow, the liquid growth medium is pumped through the developing vessels. This stretches the vessels, and the cells within them, cyclically, ensuring the production of the structural proteins needed to form a blood vessel. At the completion of the bioreactor culture, the engineered blood vessels are ready for testing and, hopefully one day, will be suitable for implantation into patients.
Dr Pashneh-Tala also utilises his Form 2 (SLA) printer to manufacture components for his bioreactors and has recently begun to produce complete chambers using this method. By doing this, he is able to quickly iterate designs based on the tissue he is creating and print components in his lab in a matter of hours, saving costs and weeks in development time.
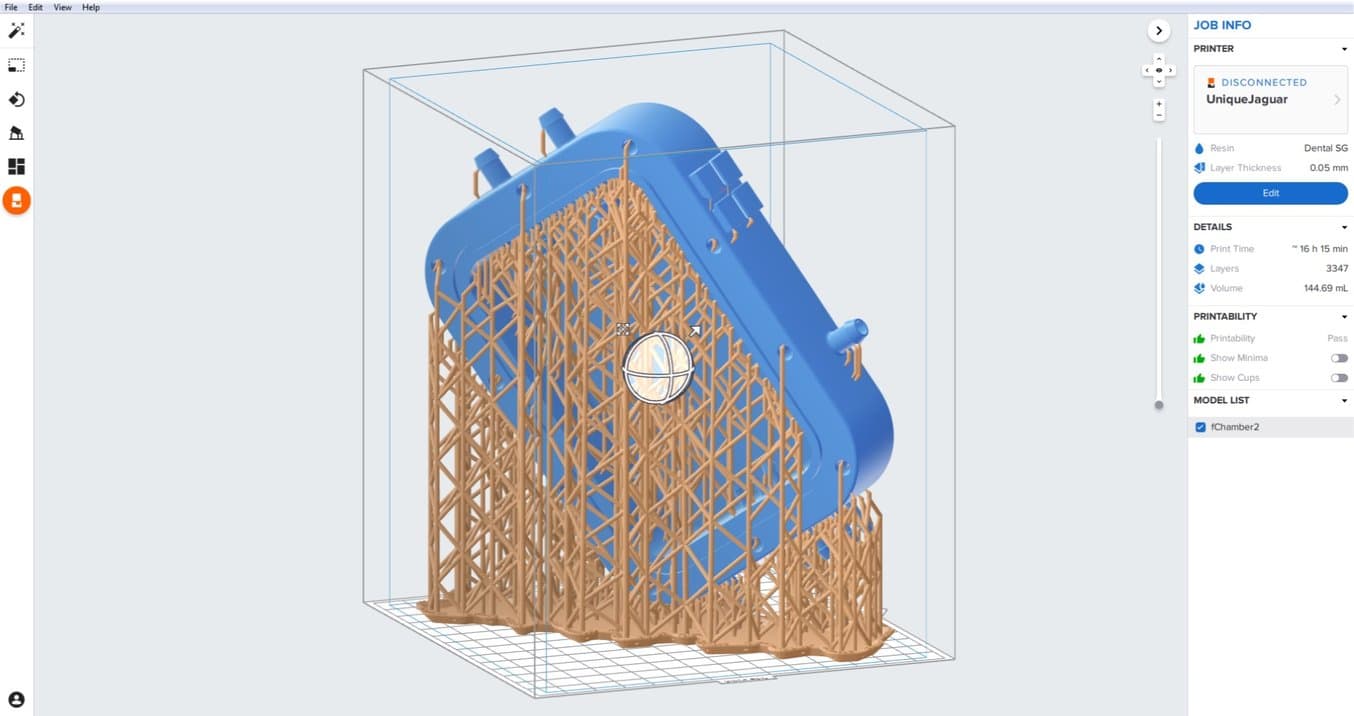
“Previously, I would have paid between £270-£300 to outsource the manufacture of a polycarbonate bioreactor chamber. With my Form 2, I am able to print the design in my lab using Dental SG, a biocompatible and autoclavable material, for £30-£40. The chamber is then easily sterilised and ready to be used straight away.”
Dr Sam Pashneh-Tala, Research Fellow, The University of Sheffield
Access to desktop manufacturing also enables Dr Pashneh-Tala to collaborate internationally on the design of his bioreactors. Working with Aptus Bioreactors, who are experts in the design and manufacture of tissue engineering bioreactor systems, Dr Pashneh-Tala is developing a system for growing tissue-engineered vein valves to provide a revolutionary treatment for chronic deep venous insufficiency.
Although Aptus are based in South Carolina and Dr Pashneh-Tala’s lab is in The University of Sheffield, they both use Formlabs desktop 3D Printers within their research facilities. This allows bioreactor components to be designed, 3D printed and tested by Aptus and then these designs shared digitally with Dr Pashneh-Tala for printing in his lab. Dr Pashneh-Tala is then also able to test the components, redesign as needed and send these digitally back to Aptus. This streamlined approach harnesses digital manufacturing capabilities and enables seamless collaboration and the rapid iteration of designs across two continents.
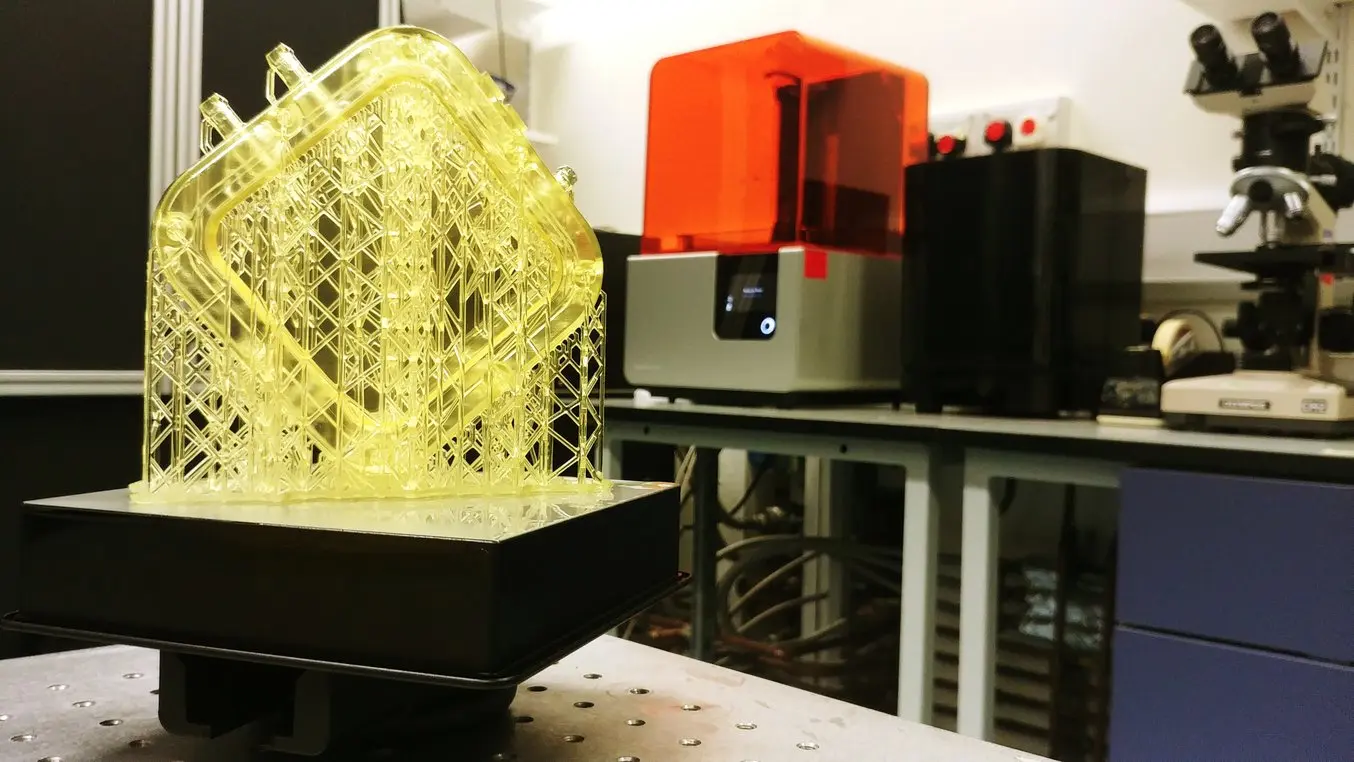
“The world is becoming smaller and more connected all the time. Increased access to digital manufacturing technologies, like 3D printing, is transforming how groups interact and collaborate on projects. By co-designing components, sharing digital files for printing locally, and testing as needed, each group can focus on the tasks they are most-suited to fulfil. This has saved Aptus Bioreactors precious time and money in shipping components between multiple locations and enabled collaborations and development that otherwise could have been too cost-restrictive.”
Lee Sierad, Founder & President, Aptus Bioreactors
Dr Pashneh-Tala’s vision is to deliver the rapid and reliable production of bespoke tissue-engineered vascular grafts designed to fit each individual patient. These designs will be generated using clinical scanning data and then manufactured using scaffolds and bioreactors produced using 3D printing technology. The tissue-engineered vessels will be produced using cells taken from the intended recipient, thus avoiding the possibility of rejection by the immune system after implantation.
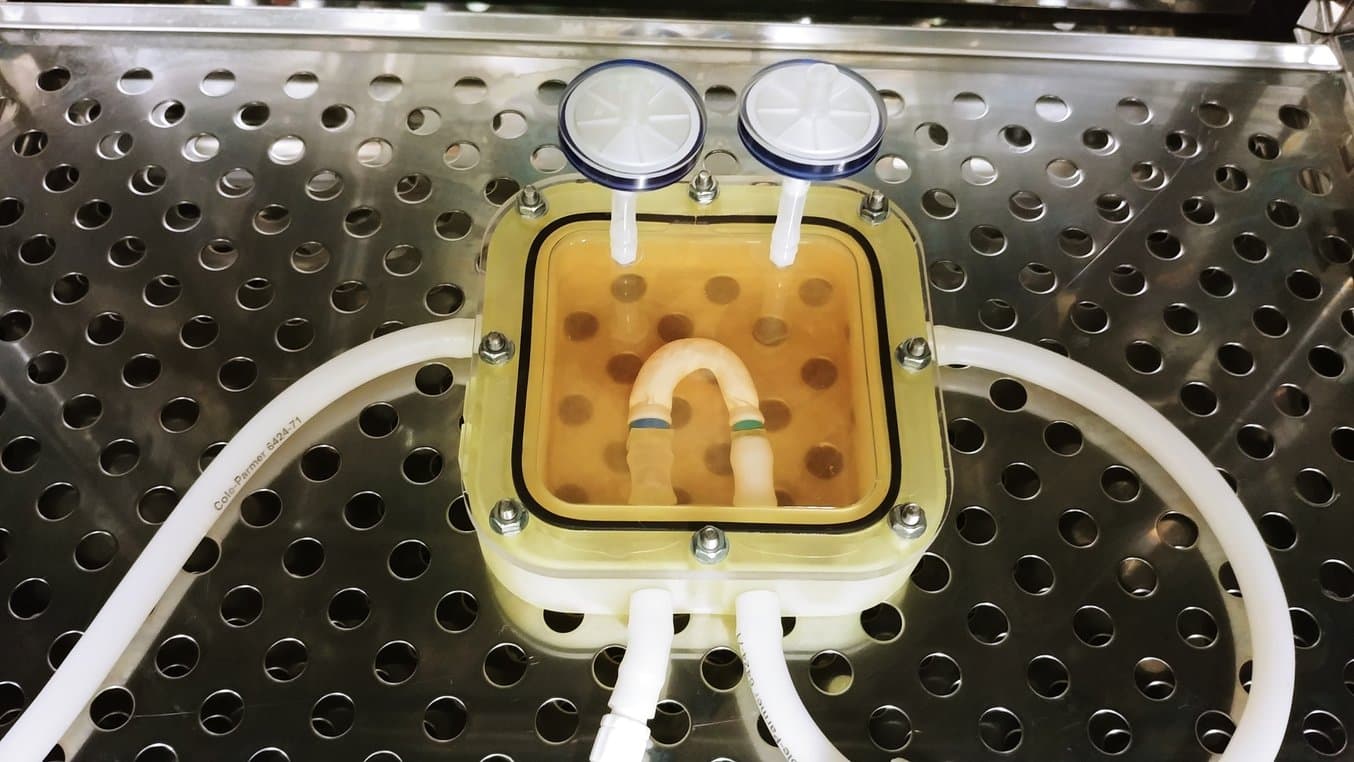
The Future of 3D Printing Medical Devices
While bioprinting fully functional internal organs such as hearts, kidneys and the liver still require more time, advancements with hybrid 3D printing techniques are happening at a very rapid rate. Precise, affordable SLA printing technologies are democratizing the research phase, allowing custom devices to be quickly manufactured and new clinical solutions developed. Increasing access to high-quality 3D printing production methods will also enable the delivery of these new treatments across the world.
“3D printing will be a key technology in the future of healthcare, assisting in the delivery of personalised medicine. Everyone’s body is different and the versatility of 3D printing makes ideally suited to accommodate this variation. The quality, economy and range of materials available through Formlabs allows me to develop solutions like never before.”
Dr Sam Pashneh-Tala, Research Fellow, The University of Sheffield
Formlabs' 3D printing systems have been used and discussed in various research projects in the scientific, medical, and dental communities. Find out more about medical applications of 3D printing, or request a free 3D printed sample to evaluate the quality of SLA printing firsthand.