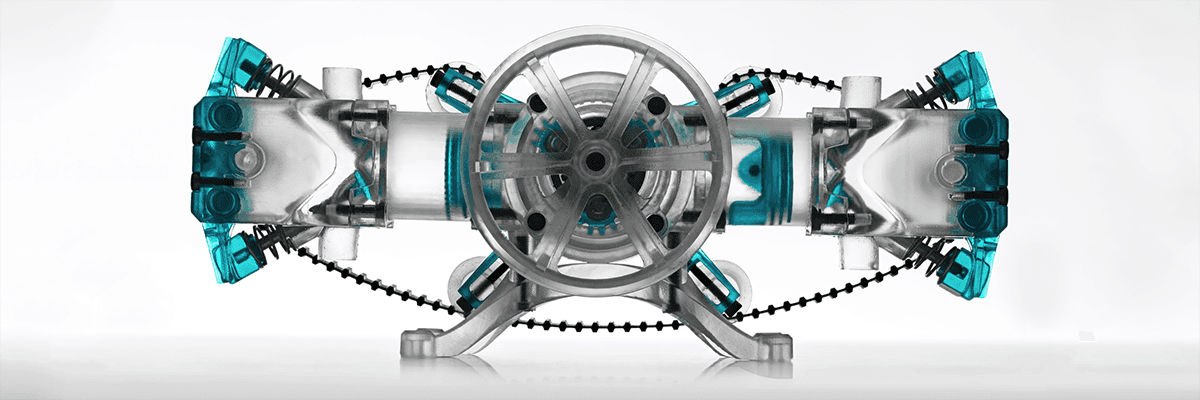
Tolerance and fit are essential concepts for any engineer designing mechanical assemblies.
Accounting for tolerances ultimately optimizes both the prototyping and production processes, reducing the material cost of iteration, lowering post-processing time, and mitigating the risk of accidentally broken parts.
In traditional machining, tighter tolerances are exponentially related to increased cost. Tighter tolerances require additional and slower machining steps than wider tolerances, so machined parts are designed with the widest tolerances allowable for a given application.
Unlike machining, additive manufacturing and 3D printing have a single automated production phase. Tighter 3D printing tolerances may require more effort in the design stage, but can yield significant savings in time and costs in prototyping and production.
In this post, we'll establish when tolerancing is most valuable for 3D printing. Then, we’ll walk through the different engineering fits to describe the basics of clearance, transition, and interference fits and when it makes sense to choose each for an assembly design.
Download our free white paper for a breakdown of how to measure and apply tolerances for each type of fit, with specific recommendations for Formlabs Tough Resin and Durable Resin.
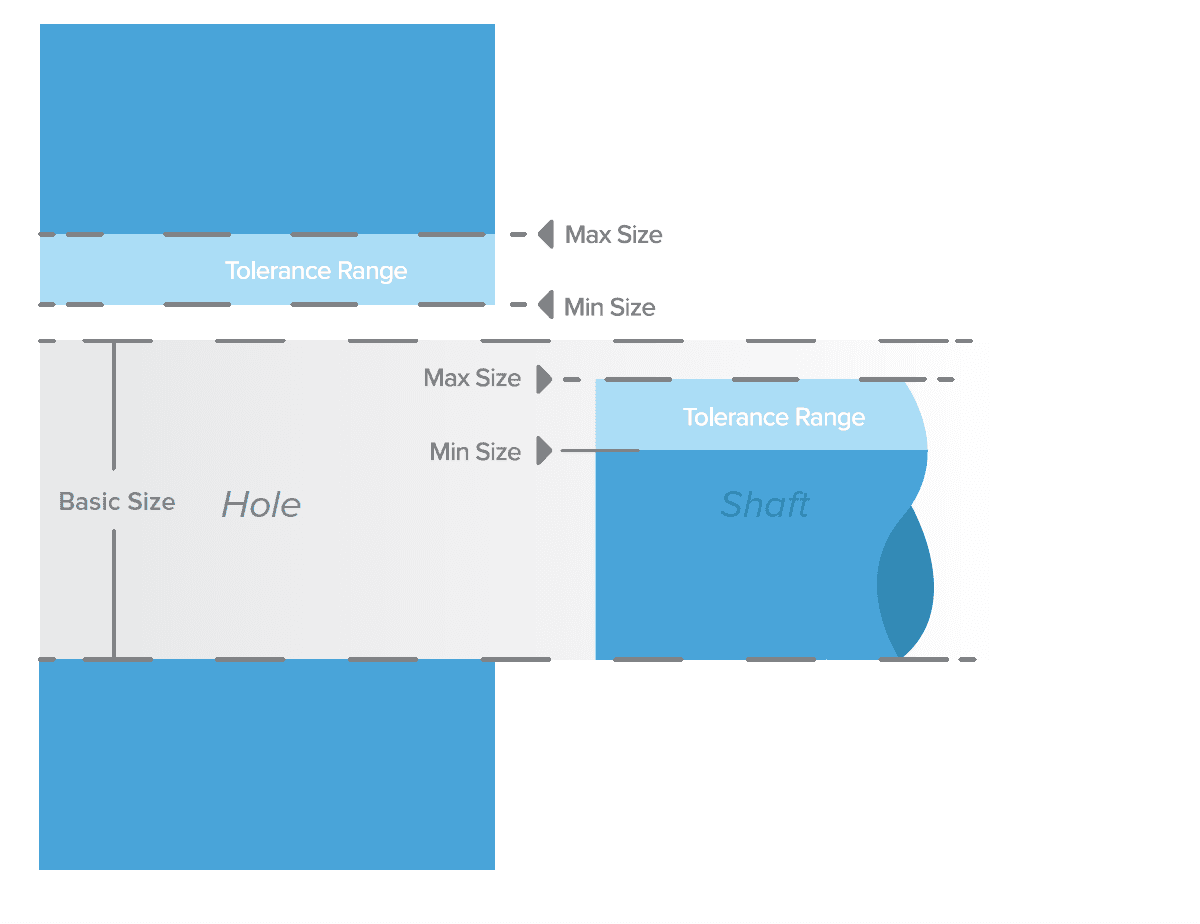
Tolerance is the predicted range of possible dimensions for parts at the time of manufacture.
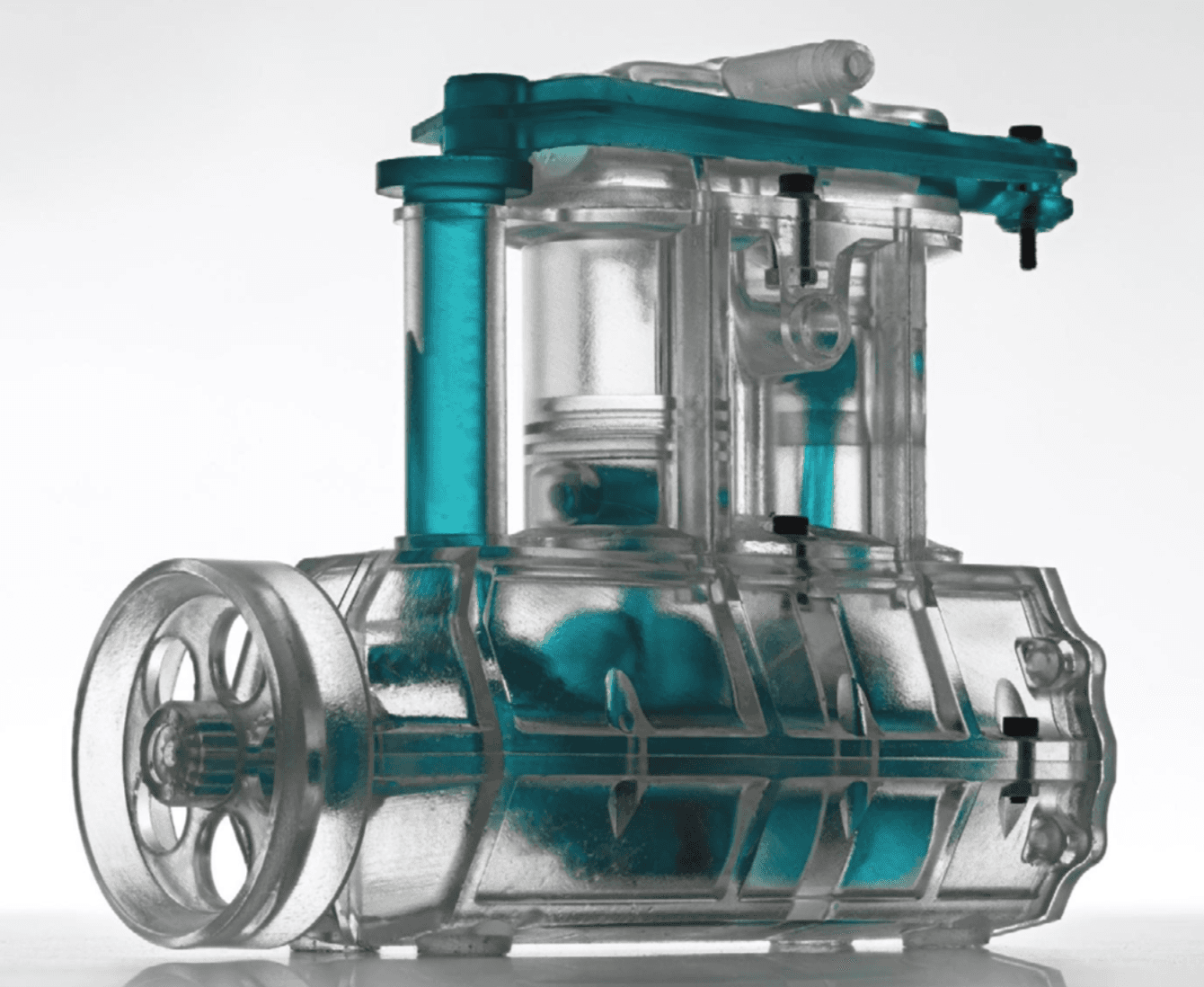
Optimizing Design for Functional 3D Printed Assemblies
SLA 3D printing offers a wide range of engineering materials, from high temperature resin for moldmaking to resin designed to withstand high stress and strain or resist wear over time. Download our white paper for specific recommended design tolerances.
Download the White PaperTolerancing for 3D Printed Large Assemblies and Small Scale Manufacturing
The static cost-per-part for 3D printing makes it a cost-effective method for prototyping and small scale manufacturing, especially for custom parts that would otherwise require significant investment in molds.
Post-processing steps for 3D printed parts assemblies include cleaning, sanding supports, and lubrication. Sanding an active surface is a reasonable method for achieving the correct fit if the part is a one-off, because less tolerancing work is required in the design phase. With larger assemblies, or when producing multiples of something, proper dimensional tolerancing quickly becomes worthwhile.
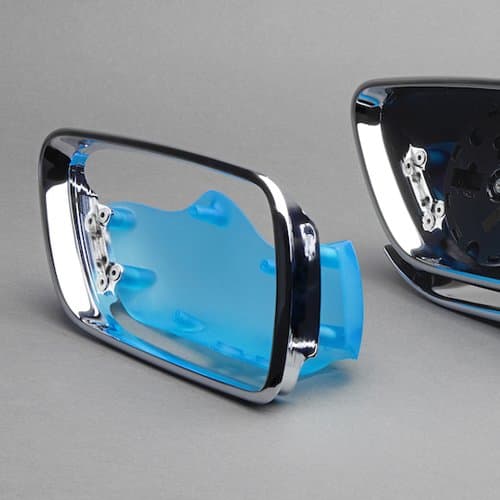
Step by Step Example: How to 3D Print Parts with Demanding Tolerances
Want to learn how to use 3D printing for engineering applications? Watch our webinar for a walk through the stereolithography (SLA) 3D printing workflow, material options, and expert tips to optimize the part-to-print workflow to get the most value out of 3D printing.
Watch the Webinar NowChoosing the Best Type of Engineering Fit
In order to understand and design the optimal 3D printing tolerances, it’s important to determine which type of fit works best for your assembly.
The functional needs of your assembly define how parts should fit together.
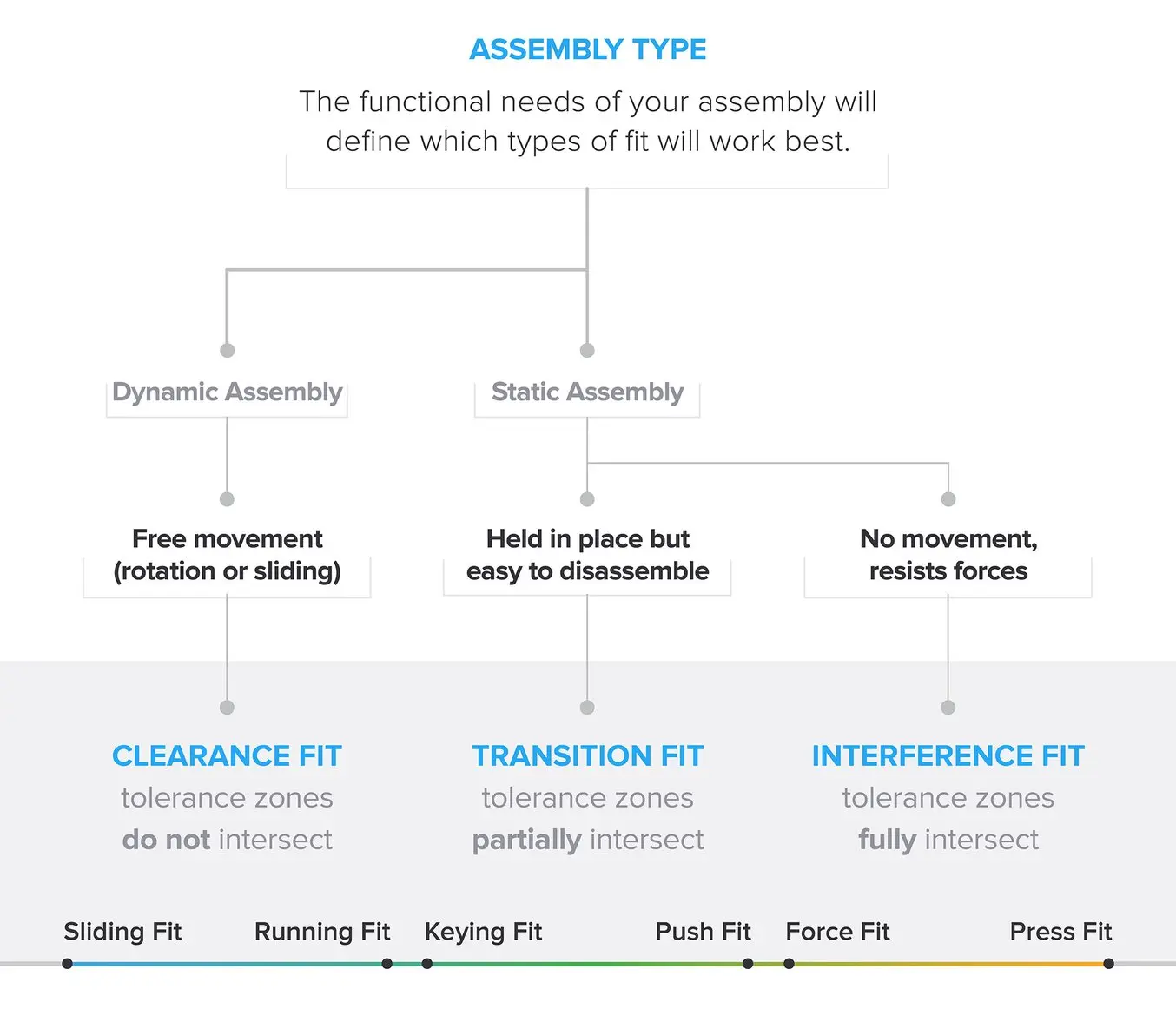
Engineering fit can be divided into three types: clearance, transition, and interference. Each of the these types of fit can then be broken down into two major subcategories.
There will always be some variation in tolerances for different manufacturing methods and depending on the 3D printing process, which means that fit is a continuum rather than completely separate stages. For example, larger clearance fits trade precision for freedom of movement. Tighter transition fits are stronger, but cause more wear on the connection. An interference fit that requires more force to join will be more challenging to disassemble.
Clearance Fit
Free movement of a component requires clearance, or space between the active surfaces. Achieve clearance by ensuring that the tolerance zones of the active surfaces do not overlap.
An active surface is a model region where two surfaces touch and either move against each other or have a static fit.
Subcategories
- A sliding fit has some lateral play, while a running fit has almost no play.
- A running fit has slightly more friction, but more accurate motion.
Play is the amount of space for movement in an unintended direction within a mechanism.
Transition Fit
If no motion between parts is needed, a transition fit allows for easy assembly and disassembly. A transition fit has partially overlapping tolerance zones.
Subcategories
- With a keying fit, a component accurately inserts into or around another part, with only a light force needed to install and remove it.
- A push fit requires more force to join and remove the parts, but they can be connected by hand.
Interference Fit
An interference fit provides a rigid, strong connection, but requires much more force applied in assembly. Tolerance zones fully intersect in interference fits.
Subcategories
- A force fit requires substantial force to install, likely with additional hand tools like a hammer, and is intended to be permanently joined.
- A press fit needs much more force to install, applied by an arbor press or similar tool.
Design Successful 3D Printed Assemblies
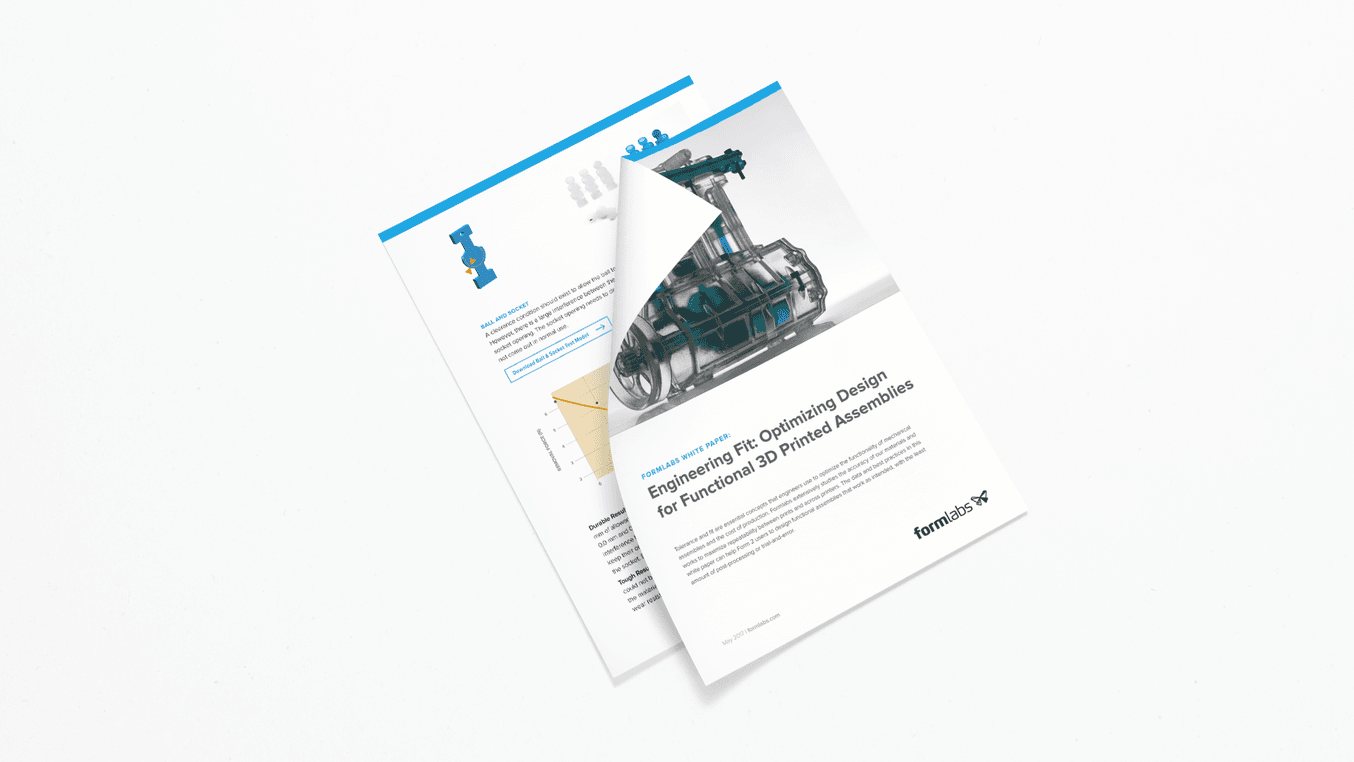
Fit ranges for common geometries can be broadly applied to many designs. Once you know the best fit, you'll need to select materials and design tolerances for your application. Our white paper, “Engineering Fit: Optimizing Design for Functional 3D Printed Assemblies,” was written to help guide these decisions.
Download the white paper for a breakdown of how to measure and apply tolerances for each type of fit, with specific recommendations for Formlabs Tough Resin and Durable Resin. The resource also includes links to downloadable test models and suggestions for lubricants, bonded components, and machining.