Imaging phantoms are objects that are a representative analog to human tissue when viewed under X-ray, computed tomography (CT), magnetic resonance imaging (MRI), ultrasound or nuclear imaging. They are used primarily for quality assurance, patient specific treatment planning, clinical training, educational, airport security and research purposes.
Conventional phantom costs are highly variable (350 - 75,000 USD)1. Phantom product accessibility is often limited by both the high cost and generality of phantom design. 3D printing has the potential to escape those limitations. Printing in-house can directly impact availability, customization and flexibility to provide clinical and educational tools based on patient population and physician needs.
3D Printing of Customizable Phantoms to Replace Cadaveric Models in Upper Extremity Surgical Residency Training
Introduction: “We designed an upper extremity phantom to address orthopedic management of distal radius fractures (DRF), a procedure typically utilizing cadaveric models…. Any model used to demonstrate this procedure must exhibit behavior sufficient to replicate that of a live patient. We do not know of any low-cost commercial DRF models with sufficient features,... The range from low-end to top-spec phantoms is quite wide, with some costing hundreds of dollars and others multiple thousands, while remaining inadequate for their intended purpose without significant modifications… We wished to improve upon these attempts by working directly with surgeons to integrate clinically important features, while utilizing low-cost materials to maintain advantages over both commercial phantom and cadaveric models.”
Materials and Methods: “For our models to exhibit the same sensory feedback surgeons expect from true fractures, we captured and translated the mechanical requirements of DRF reduction into phantom components: (1) a periosteum-like sheath on the bones to improve cartilaginous joint behavior, (2) better replicate the behavior and difficulty of manipulating a real patient by creating an entire arm from the phalanges to the proximal humerus, (3) incorporate the use of radiopaque elements to allow for medical imaging of surgical accuracy, and (4) an opaque covering to mimic skin and restrict visual cues from the phantom’s internal structure.”
"In order to create an opaque skin covering the arm model shape was printed directly to create an overmold. Dyed rubber is then cured on-to the surface printed with Formlabs resin to create, in essence, a glove for the phantom."
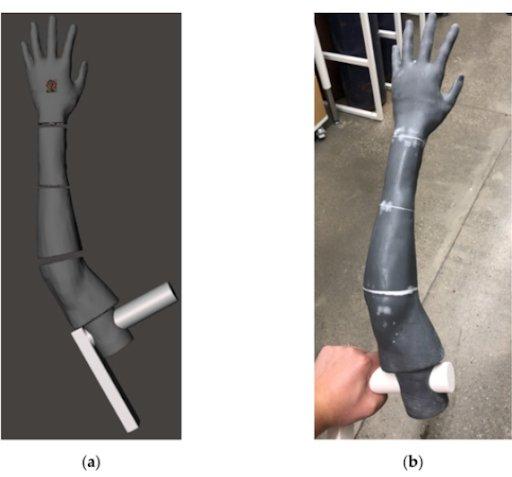
(a) phantom arm geometry (gray), with grip (white), in Meshmixer. (b) Skin covering overmold printed in parts with a Formlabs resin.
Another mold was created to have a full arm and not just the respective bones. Ballistic gelatin was used for this step, which must be heated above 275 °F (135 °C) to melt. “The mold pieces are printed using stereolithographic (SLA) Formlabs Form 3 resin printers (FormLabs, Somerville, MA, USA), which create smoother and more temperature-resistant prints than those produced by FDM”
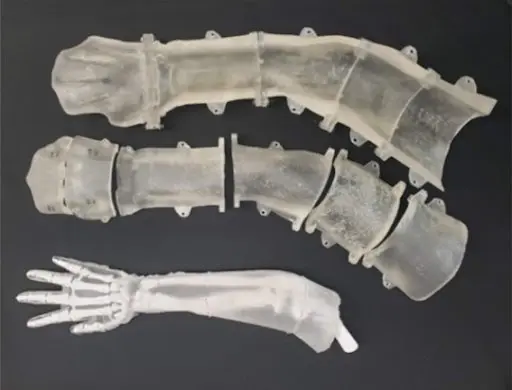
Photograph showing an unskinned phantom arm (bottom) beside its multi-piece casting mold (top) printed from a FormLabs’ SLA resin.
Results and Discussion: “Twenty early (post-graduate years 1 and 2) upper extremity surgical residents… performed DRF management on our phantoms across two sessions”
“The work here in creating relevant clinical features in a DRF phantom shows that cadavers are replaceable for early resident skill training. The training was completed successfully for all participants, demonstrating the phantom’s ability to convey required stimuli normally found from live patients or cadaveric models. Interactive training with synthetic phantoms allows for attending surgeons to evaluate resident performance and grade the application of classroom techniques in a safe, practical, and low-cost environment. As well, the residents indicated their strong approval for this type of learning activity, expressing a willingness to repeat the training or participate in future trials. This internal motivation is key to long-term engagement and integration of component skills, contributing more broadly to increased quality of care for patients”
Conclusion: “This orthopedic surgical training and assessment procedure, traditionally relying on cadaveric models, was successfully completed using synthetic phantoms. Students were able to successfully complete a radial reduction procedure utilizing prior classroom knowledge and enjoyed the opportunity to apply prior classroom knowledge in an active setting.”
“This control over replica anatomical features offered by phantoms cannot be matched in cadaveric samples, enhancing training through repeatability and standardized scoring.
The accuracy and rapid modification of our phantoms is only possible through the fine detailing and rapid production nature of additive manufacturing.”
Computed tomography tissue equivalence of 3D printing materials
Introduction: “The aim of this study was to determine the CT number values of commercially available fused deposition modeling (FDM) and stereolithography (SLA) AM materials.”
“Fabricated AM anatomical models are useful for creating dose optimization anthropomorphic phantoms in diagnostics as radiology as well as in radiotherapy for creating patient-specific phantoms that can be used in quality assurance or treatment plan evaluation in anatomically challenging or dosimetrically complex cases.”
Materials and Methods: “Total of 15 AM materials, seven FDM and eight SLA, were selected and scanned on CT to determine the HU value and appearance on the images.” All test objects were designed as rectangular blocks and after their production physical descriptions were calculated. AM materials were scanned on CT operating at 80, 100, 120 and 135 kV.”
The designed SLA-cubes were printed on Form 3B.
Results: “All materials correspond to a certain human tissue and they have uniformity when printed with 100% infill. CT number ranged… from −15.8 HU to 167.3 HU, for SLA materials.”
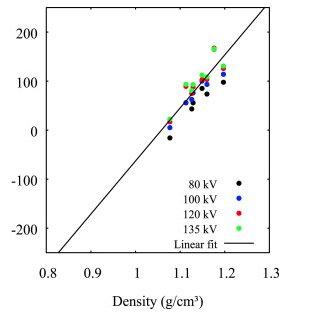
This figure represents the relationship between sample SLA materials density and CT number (HU). Higher material density is associated with higher CT numbers.
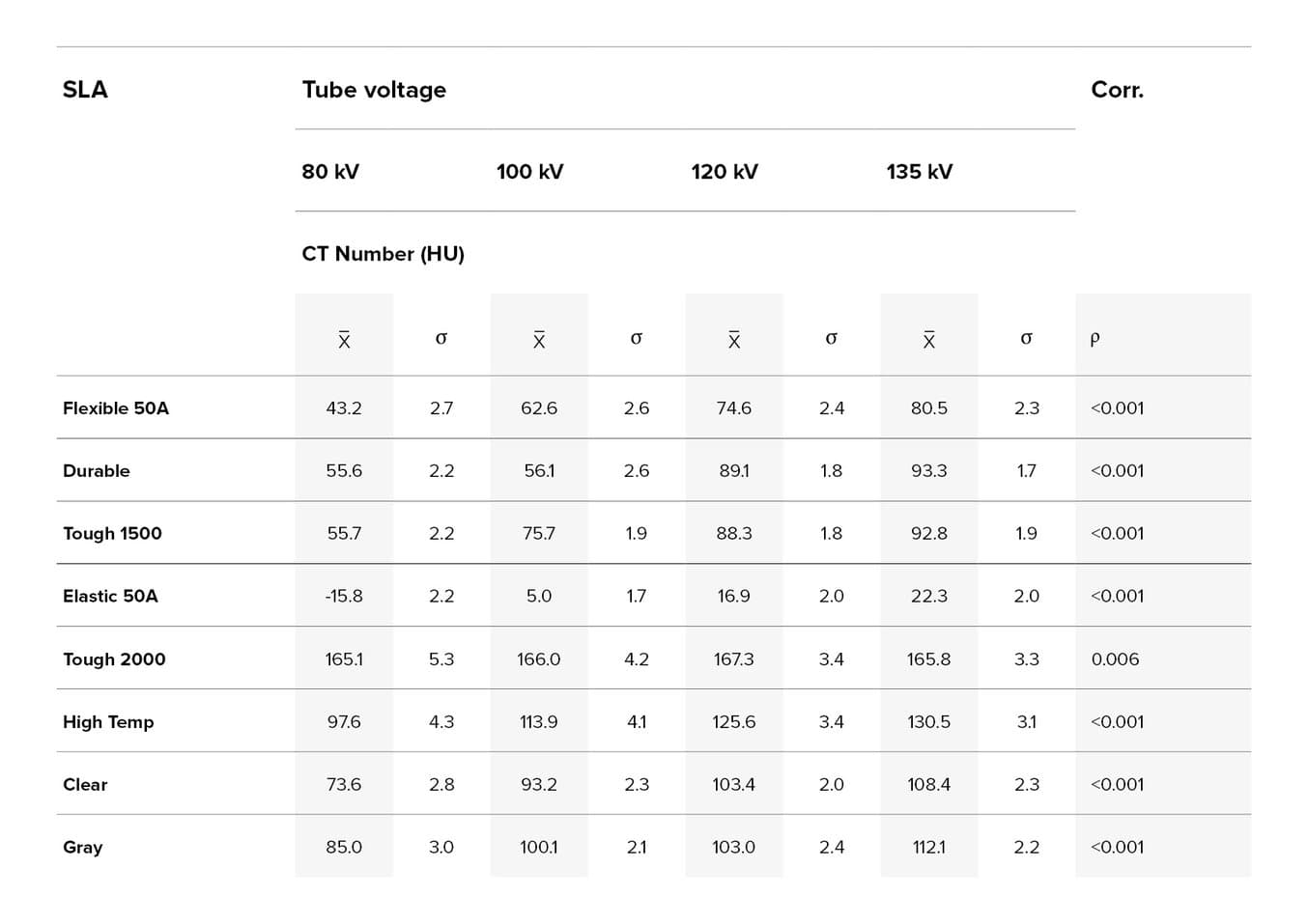
Average (x) and standard deviation (σ) of CT numbers measured in samples of materials used in stereolithographic (SLA) technique of additive manufacturing (AM), and p value of Kendall's τ correlation test between average CT number and tube voltage (kV).
Some AM materials have a HU value very similar to the number of human tissues,… and can therefore be used to develop anatomically accurate phantoms produced from CT scans using AM. In the table below, CT numbers expressed in terms of Hounsfield units for different human tissues and organs.
Tissue | Hounsfield Unite (HU) Scale |
---|---|
Air | -1005 to -95 |
Lungs | 950 to -550 |
Fat | -100 to -80 |
Water | 4 to 4 |
Kidney | 20 to 40 |
Pancreas | 30 to 50 |
Blood | 50 to 60 |
Liver | 50 to 70 |
Spongious Bone | 50 to 300 |
Compact bone | 300+ |
Discussion: “Knowing the CT number of an AM materials can allow the design of medical or rehabilitation products with a specific appearance on CT images. Analyzed and collected data can find application in the design and manufacture of immobilization devices that can be easily distinguished from other materials or human tissue.”
This study shows that there is significant potential for the use of AM material for sophisticated test objects when used in medical image modality testing. By using this approach it allows us to optimize parameters of CT scanners for better image quality and lower radiation dose with anthropomorphic phantoms that mimic different structures. Some AM materials have a HU value very similar to the number of human tissues,... , and can therefore be used to develop anatomically accurate phantoms produced from CT scans using AM.
Implications for practice: “Knowing actual CT numbers of frequently used AM materials allows manufacturing anthropomorphic phantoms to investigate radiation doses in diagnostic radiology and radiotherapy.”
Training Ultrasound Image Classification Deep-Learning Algorithms for Pneumothorax Detection Using a Synthetic Tissue Phantom Apparatus
Introduction: ”Ultrasound (US) imaging is a critical tool in emergency and military medicine because of its portability and immediate nature. However, proper image interpretation requires skill, limiting its utility in remote applications for conditions such as pneumothorax (PTX) which requires rapid intervention. Artificial intelligence has the potential to automate ultrasound image analysis for various pathophysiological conditions. Training models require large data sets and a means of troubleshooting in real-time for ultrasound integration deployment, and they also require large animal models or clinical testing. In response, here we detail the development of an inexpensive, simple method to create a PTX US-compliant phantom for the primary purpose of the preliminary development and troubleshooting of deep learning algorithms.”
Materials and Methods: “The model comprises a synthetic gelatin phantom cast in a custom 3D-printed rib mold and a lung mimicking phantom.”
“A two-piece mold was developed based on measurements of a portion of the human thoracic region, … and was fabricated using either stereolithographic printing with High Temp Resin (Formlabs, Somerville, MA, USA) or filament deposition modeling with co-polyesters… ”
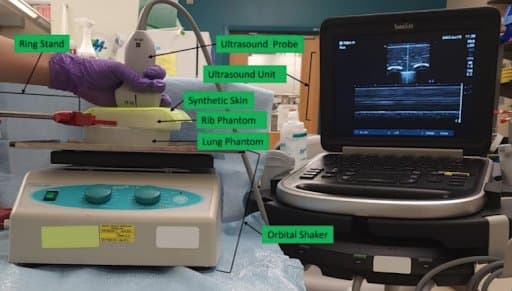
Image acquisition platform incorporating (top to bottom): ultrasound probe manually held in place and connected to the ultrasound unit, synthetic skin positioned atop the rib phantom that was held in place by the ring stand, and the lung phantom located on the bed of the orbital shaker.
Images from the phantom were compared to images from swine. “We then used a deep learning image classification algorithm, which we previously developed for shrapnel detection, to accurately predict the presence of PTX in swine images by only training on phantom image sets, highlighting the utility for a tissue phantom for AI applications.”
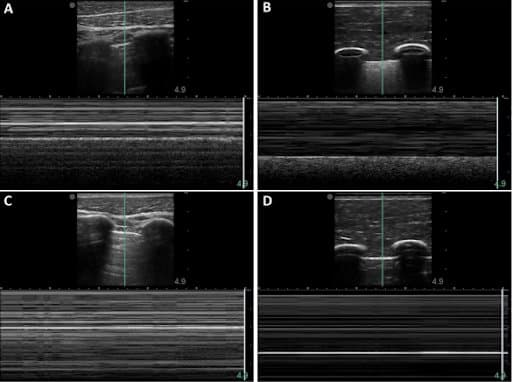
When the M-mode line was centered in the pleural lining, the baseline porcine subject provided the typical “sea-shore” sign (A). The post-injury porcine M-mode image lost this appearance and instead presented a “stratosphere sign”, indicating a lack of lung sliding (C). The synthetic phantom produced similar “sandy beach” characteristics (B) when motion was functional on the apparatus, and it produced the “barcode” when motion was halted (D).
Results: “When compared to PTX images acquired in swine, images from the phantom were similar in both PTX negative and positive mimicking scenarios.”
“Next, we evaluated how the image sets acquired from the PTX phantom apparatus could be deployed for deep learning algorithm development… the trained model was 100% accurate on classifying positive/negative PTX images from the synthetic phantom apparatus. For a more realistic testing approach, swine PTX M-mode images were evaluated by the model.... resulting in 99.8% true positive and 87.3% true negative swine test accuracy, with an overall accuracy of 93.6%” (when multiple augmentation methods were applied).
Discussion: The PTX phantom was simple to configure, relying on 3D-printed parts and synthetic ballistic gelatin. The resulting images were similar to swine images for both B-mode and M-mode ultrasound data captures. The phantoms were shelf stable, allowing for repeated use, and, by automating the lung motion, a wide range of respiratory rates was possible…. Thus, a simple PTX phantom apparatus such
as this can reduce the extent of swine or human image collection requirements, which may be needed for PTX imaging applications. It does not replace the need for live data collection, but initial troubleshooting, training, and algorithm development may be possible with a synthetic phantom apparatus.
Conclusion: In conclusion, this synthetic phantom apparatus development addresses a current gap in the generation of large image datasets for deep learning algorithm development.
Development and Proof of Concept of a Low-Cost Ultrasound Training Model for Diagnosis of Giant Cell Arteritis Using 3D Printing
Introduction: ”Currently, ultrasound (US) is widely used for the diagnosis of giant cell arteritis (GCA). Our aim was to develop a low-cost US training model for diagnosis of GCA of the temporal and axillary artery using a modern 3D printing system.”
Materials and Methods: “We designed an US training model, which enables measurement of the intima-media thickness (IMT) of temporal and axillary arteries using Autodesk Fusion360. This model was printed using a modern 3D printer (Formlabs Form3) and embedded in ballistic gelatine. The ultrasound images including measurement of the IMT by ultrasound specialists in GCA were compared to ultrasound images in acute GCA and healthy subjects.”
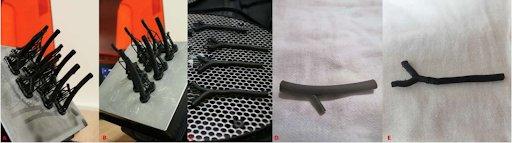
Manufacturing process of the temporal artery models at various stages. (A) Picture taken just after the printing process finished of an axillary artery model. (B) Image after washing of the axillary artery models in isopropanol. (C) Picture after hardening of temporal artery models with 405 nm LED light. (D,E) high resolution images of a temporal and axillary model respectively.
Results: “Our ultrasound training model of the axillary and temporal artery displayed a very similar ultrasound morphology compared to real US images and fulfilled the OMERACT ultrasound definitions of normal and pathological temporal and axillary arteries in GCA. The IMT measurements were in line with published cut-off values for normal and pathological IMT values in GCA and healthy individuals. When testing the models on blinded US specialists in GCA, they were identified correctly in all test rounds with an intra-class coefficient of 0.99.”
Conclusion: “The production of low-cost ultrasound training models of normal and pathological temporal and axillary arteries in GCA, which fulfill the OMERACT ultrasound definitions and adhere to the published IMT cut-off values in GCA, is feasible. Ultrasound specialists identified each respective model correctly in every case.”
Radiological Properties of Formlabs Resins
We’ve conducted a study into the radiological properties of Formlabs resins in conjunction with the University of Florida Health - Jacksonville. The results demonstrated that, for CT imaging, many of Formlabs resins have Hounsfield Unit densities that are within 1 standard deviation of known human tissue. The following are images and density results produced during that initial study.
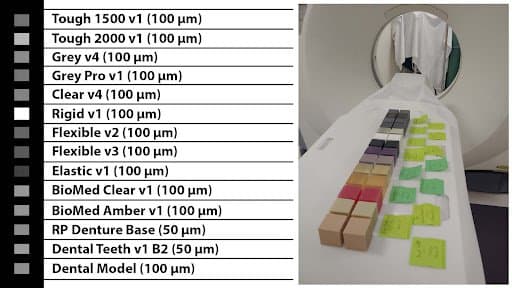
The resins chosen in this initial study did not show substantial differences in magnetic resonance imaging or for ultrasound imaging.
Formlabs resins don’t have the necessary free hydrogen protons to image well on an MRI, and therefore have very low signal and display as black. That said, the study did note that Formlabs resins do not produce artifacts commonly seen with imaging metals and other dense materials.
Resin | Mean | SD | ||
---|---|---|---|---|
Tough 1500 | *80.475 | 10.15 | ||
Tough 2000 | 151.5 | 10.675 | ||
Grey V4 | *108.425 | 10.275 | ||
Grey Pro V1 | *85.025 | 10.1 | ||
Clear V4 | *102.825 | 10 | ||
Rigid V1 | 334.55 | 12.175 | ||
Flexible V2 | *66.425 | 9.9 | ||
Flexible V3 | *50.625 | 9.675 | ||
Elastic V1 | 21.45 | 9.375 |
Note: Data is for materials tested at Averaged HU Soft 5mm. For the full results, along with additional materials, watch the video about our report into the radiological properties of Formlabs resins .
In the case of ultrasound imaging a recommendation was given to fill hollow, thin-walled printed structures with water or gel for effective imaging (because of the acoustic interference between cured resin and surrounding materials).
All tested Formlabs materials demonstrated expected and reproducible characteristics with CT, MRI and US imaging.
The tested materials created no imaging artifacts.
Conclusion: For clinical applications, knowing that a material has a certain imaging signature, or does not create artifacts is vital in giving an accurate diagnosis with minimal uncertainty.
With their known mechanical and medical imaging characteristics, many resins are suited in creating bones and vascular or soft tissue structures for simulation models. If a resin material is used in future research, the properties of the material are known and no longer become a ‘variable’ which lowers the power needed to obtain statistical significance.
Topic Summary
In this paper we have presented independent publications with Formlabs material. As demonstrated above, SLA technology has enabled the creation of affordable realistic upper extremity phantoms to replace cadaveric models in surgical residency training, and of low-cost ultrasound training models of arteries to enhance practical education of rheumatologists and radiologists worldwide. It can also reduce the need for large animal models or clinical testing by producing an inexpensive ultrasound-compliant phantom for the preliminary development of deep learning algorithms. Finally, assessing the radiological properties of Formlabs resins with different imaging modalities provides valuable data for more accurate diagnostics, future research and the manufacturing of anatomically accurate phantoms and immobilization devices for several clinical applications.